by Michael Palmer, MD
Karl Popper
This article was written as a rebuttal to a piece by German journalist Torsten Engelbrecht [1], which in turn was a reply to my own piece (co-authored with Sucharit Bhakdi) [2]. Engelbrecht’s arguments were a subset of the familiar no-virus narrative; therefore, this reply may be of interest to others.
1. What actually is “scientific proof?”
Most of the arguments that we hear from Torsten Engelbrecht and from the no-virus camp as a whole boil down to the claim that “there is not enough evidence.” For example, Engelbrecht says right at the beginning of his piece that “the so-called ‘germ theory’ is based on unproven assumptions.” Further down he states that “no solid control experiments have been carried out” etc.
It is therefore worth dwelling briefly on the question of what the word “proof” actually means in the empirical sciences. For me, the most compelling view is that of the philosopher Karl Popper, which he set out in his book “Die Logik der Forschung” [3] (Engl.: The Logic of Scientific Discovery). According to Popper, a scientific theory can never be definitively proven. If out of a thousand swans that we observe, all are white, then the hypothesis that all swans are white is reasonable; but the observation of a single black swan could disprove it at any time. The refutation is not quite so simple with less strict statements—for example, that only the majority of all swans are white. But the same principle still applies: a finite number of individual experimental observations will never allow us to make an absolutely certain prediction about a potentially infinite number of future individual cases.
Nevertheless, while we can never really prove a hypothesis through experiments, we can still test it; and as long as these tests do not disprove our hypothesis, we can stick to it. But how far should we take our testing? That will certainly depend on the attitude of the audience—mostly our peers, but for issues of general importance, the public at large. If we believe we have made a revolutionary discovery, then we must prepare for a skeptical audience; and we will therefore have to carry out particularly thorough and comprehensive tests to convince such an audience of our hypothesis. If, on the other hand, we only want to report some incremental progress that is generally consistent with the prevailing theory, then the audience will not expect our tests to start again with Adam and Eve, but will be satisfied with correspondingly selective and limited tests.
Based on these considerations, we can already understand some of the no-virus faction’s fallacies. The theory of viruses and other microbial infectious agents—the germ theory—has passed numerous tests since its inception in the late 19thcentury and is therefore widely accepted today. Many of these tests have been associated with successful applications; beneficial ones such as hygiene measures to prevent infections and epidemics, but also tragic ones such as the use of pathogenic bacteria as biological weapons.
Against this background, it is pointless to shout from the gallery: “You don’t have enough evidence!”—which simply means: “We demand more tests!”, because for most people, especially most experts, the number of tests that have been successfully passed is apparently enough after all. In order to shake the prevailing doctrine of infectious viruses and other germs, the virus skeptics1 would have to show what tests germ theory has actually failed, or they would have to demonstrate such failure in experiments they have carried out themselves.
Another mistake is to expect to find exhaustive discussions and experimental evidence on the question “Do viruses really exist?” in recent studies. Such fundamental discussions and evidence can only be expected in studies from an earlier period, in which the idea of viruses was still new and controversial. And of course, when looking at such earlier studies, it must be acknowledged that the experiments of each period were limited by its technical possibilities. Due to the historical development, each individual study will therefore be incomplete in some respect. Only by looking at the cumulative evidence in its entirety will one be able to form a correct picture of germ theory’s strength or weakness. And, if they want to be taken seriously, the virus skeptics would have to do this homework themselves; their expectation that others should contract miners’ lung while digging up their “proof” for them is irritating, to say the least.
2. On control experiments
Suppose I had found a new chemical compound and I thought it lowered fever. What could a minimal experimental test look like? I could first experimentally induce fever in a few mice and then administer my new wonder drug to these. If the fever then subsided, my hypothesis would have passed its first test.
Of course, no one would accept such a test as sufficient, as it is common experience that fever usually subsides spontaneously. I would therefore quite rightly be expected to compare my miracle cure with a placebo. But nonetheless, my naive experiment would also be a real test—if the fever did not subside, then I would have to reject my hypothesis even without placebo controls.
As a second example, let’s assume that I had found a new pathogenic microbe and I believed that it was always and immediately lethal after injection under the skin. So I inject a few mice with a pure culture of this germ, and after a short while they drop dead as expected. How important would it be in this case to inject a control group of mice with a sterile solution? This would be common practice, but it would certainly be less critical than the aforementioned placebo control, because experience has shown that mice do not spontaneously and simultaneously drop dead for no reason. A “sudden mouse death syndrome” is much less likely than the spontaneous resolution of a fever.
These examples are only meant to illustrate that, while control experiments are usually a good idea and can prevent us from prematurely accepting an erroneous hypothesis as “sufficiently tested”, a real or alleged lack of control experiments does not automatically invalidate the experiments in question. Controls are not a necessary or sufficient ritual to ensure that one is doing “real science.” A control experiment serves to rule out an alternative hypothesis, usually a trivial one, which could explain the same observations that I want to explain with my preferred hypothesis. It is pointless to call for “more controls” in general, as the virus skeptics like to do, without precisely naming the alternative hypotheses that would have to be ruled out in each case.
So much for the scientific method and its misuse by virus skeptics. In the following, I will address some specific arguments of the virus skeptics on germ theory and virology.
3. On Robert Koch
The germ theory of infectious diseases is tightly linked to Robert Koch and his discoveries of the causative agents of anthrax, cholera and tuberculosis. In his reply to us, Engelbrecht reduces the significance of Robert Koch to that of a scientific charlatan:
Engelbrecht presumes to disqualify Koch entirely because of this episode:
Of course, the events highlighted by Engelbrecht should not be swept under the carpet; they show that Koch, like most people, was prone to error and probably also to wishful thinking. On the other hand, Koch’s error does not disprove his discovery of the tubercle bacillus. On the contrary, the discovery of his error shows that the public was quite capable of meeting even this “hero” of science with robust criticism. It rejected the tuberculin cure because it proved worthless; but it allowed Koch’s discoveries on the cause of tuberculosis to stand because they could be successfully verified and reproduced [4]:
The cited work by Grange and Bishop [4] is well worth reading and gives a good account of Koch’s own studies, as well as the scientific questions and discussions that followed Koch’s discovery. People asked, for example, whether the tubercle bacillus was the cause or the consequence of the disease, whether tuberculosis could also exist without this bacillus, and so on. Contrary to what the critics of germ theory would have us believe, such critical questions are not new, but were immediately raised and rigorously investigated by Koch’s clear-minded and critical scientific contemporaries.
4. On the detection of viruses in general
Engelbrecht’s criticism of Koch and his bacteria is really more of a “bonus feature”—the main subject of the controversy is the existence of viruses that cause disease. Engelbrecht maintains that virus particles are really only cell fragments or subcellular structures. In our preceding piece, we had commented as follows:
Engelbrecht’s counter-“arguments”—this word really only fits here with quotation marks—are as follows:
- The CEO of a DNA sequencing machine manufacturer once told the World Economic Forum that the people at Moderna had started developing their vaccine without even having seen a live SARS-CoV-2 virus.
- Clinical samples used for the PCR detection of viruses contain a wide range of endogenous particles and molecules that have nothing to do with the virus being searched for.
Both “arguments” have no logical relationship to our statement that I can see. I will come back to SARS-CoV-2 later. With regard to the second “argument”, a fundamental misunderstanding must first be cleared up.
4.1. Diagnostic detection without purification
Virus detection by PCR is by no means the only diagnostic method in laboratory medicine that is applied to complex sample mixtures. For example, most blood tests carried out in clinical chemistry laboratories do not require prior purification of the substance to be detected. This is possible if you have a specific probe for the substance in question. There are several variations on this theme:
- In many cases, specific antibodies are used. This is widely used in the detection of infectious agents, but also of endogenous substances such as hormones. The antibodies “fish” the substance in question out of the mixture, and there are various techniques for subsequent quantitation of the “catch.”
- The activity of enzymes in the blood can be measured by supplying them with their specific substrates and then measuring how quickly these are turned over. Conversely, glucose can be detected in the blood by adding an enzyme to the sample that specifically recognizes and converts glucose.
- PCR methods are widely used not only in virology, but also in genetic counseling and forensics, for example. The specificity is based on the base pairing between the made-to-measure synthetic oligonucleotides (primers) and the nucleic acid sequence being searched for. This can be a mutant gene that is associated with a genetic risk, the chromosomal DNA of a violent offender, or viral DNA or RNA sequences. The specificity of the detection can be ensured by sequencing the PCR products. Like any tool, the PCR method can be used correctly or incorrectly. During the COVID-19 pandemic, it was mostly used incorrectly.
If we were to give in to the virus skeptics in their attempt to ban all diagnostic tests that are based on the selectivity of the diagnostic reagents and not on the complete purification of the virus to be detected, then we would consequently have to abolish the majority of all other diagnostic lab tests in medicine.
Of course, this suggestion is not meant seriously; it is merely intended to illustrate that the virus skeptics are out of touch with real life. And this applies in two senses: life as we know it is only possible through highly specific mutual recognition of biomolecules. Laboratory methods such as those just described simply take advantage of this fundamental property of life itself.
4.2. The scary tale of the exosomes
Among the various components of patient samples that allegedly prevent reliable detection of SARS-CoV-2 and other viruses in such samples, Engelbrecht also lists the so-called exosomes. These are small vesicles that bud off from the surfaces of our body cells and can then be reabsorbed by other cells. In this way, our cells can exchange various cellular components with each other.
Exosomes are particularly popular among virus skeptics; they are always called upon when a particle that looks like a virus needs to be explained away. Allegedly, so the myth goes, it is impossible to separate or distinguish viruses from exosomes. Engelbrecht writes:2
In other words, if you put the sample in question into a tube and spin it in the ultracentrifuge, you will find the cellular vesicles and the virus particles together at the bottom of the tube. That may be so, but this is not the only or the best method for purifying virus particles. Engelbrecht also admits this:
With this, we have introduced another favorite bogeyman of the virus skeptics—cell cultures, which cannot be trusted because they contain exosomes that could be mistaken for viruses. Here the reader might wonder: If both patient materials and cell cultures are replete with exosomes, why should we trust a virus preparation from a patient material but reject one from a cell culture? Shouldn’t we either reject both or accept both?
Be that as it may—let’s turn the tables and test the idea that virus particles are really just cellular exosomes. What predictions follow from such a hypothesis? Off the top of my head, I can think of these two:
- If we inoculate the same kind of cell culture with two or more different viruses, we should still only ever get back one and the same “virus”—because this “virus” would be a mirage, and its particles only the products of the cultured cells themselves.
- Since the introduction of cell cultures, there should no longer be any diseases of unknown cause. After all, every such disease—and especially every inflammatory disease—has certainly been suspected of viral causation at least once; and the inoculation of a cell culture with the corresponding patient samples would have immediately led to the detection of exosomes, which would then have been presented as the particles of the supposed causative virus.
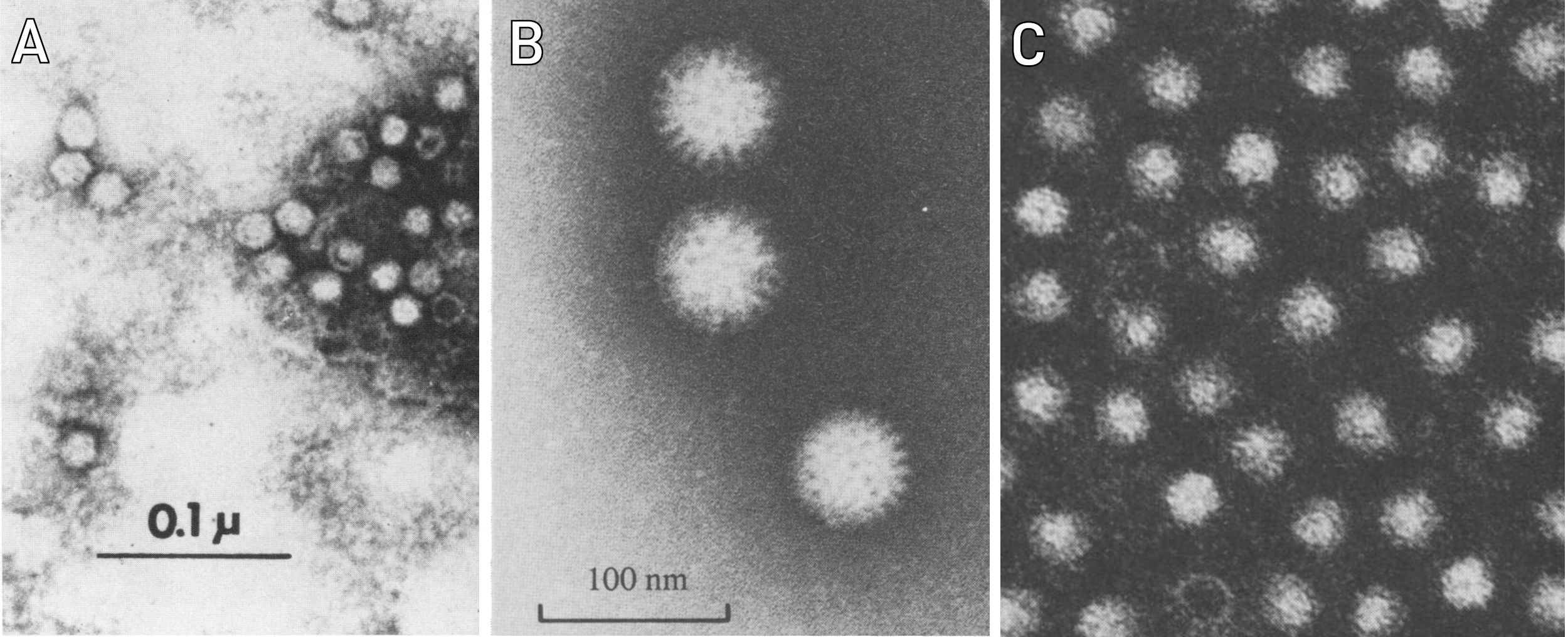
As to Point 1: Figure 1 shows electron micrographs of three different viruses grown in similar cell cultures. The reader may judge for himself whether these three viruses all look the same or not. As to Point 2: Finding diseases with still unknown causes is left as an exercise to the reader. Inflammations of the central nervous system are a fruitful area of inquiry. The failure of countless attempts to identify causal viruses for such diseases shows, of course, that virologists are very well able to distinguish virus particles from exosomes or other cellular components. This hypothesis of the virus skeptics can therefore be considered unambiguously falsified.
A few more comments on the subject of electron microscopy: this method, too, is subject to fundamental criticism by virus skeptics. An anonymous online article [8], which like Engelbrecht’s piece was written in response to our own previous essay, quoted a historical report on virus research [9] as follows:
In the present context, I could imagine that the virus skeptics will now completely denounce electron microscopy as unreliable in order to evade falsification of their cherished exosome hypothesis. I therefore want to point out that the above quote dates back to the Stone Age of electron microscopy, so to speak, and that the problem it alludes to was solved a short time later.
Images like the one in Figure 1 are taken using the “negative staining” technique. Here, the biological material is not imaged directly. Instead, it is first coated with an inorganic contrast agent. This creates a cast, a kind of “death mask” of the biological material. It is this mask that then appears in the image, and it easily withstands the effects of the high-energy electron beam.
A more modern solution to the same problem is known as cryo-electron microscopy. This involves freezing the sample at a temperature close to absolute zero. In this state, it is less sensitive to structural damage caused by the electron beam. Modern, high-resolution electron detectors allow more detailed imaging, which is increasingly making molecular structures accessible for examination.
4.3. On the purification and characterization of viruses from patient materials
Another of Engelbrecht’s daring theses is as follows:
As discussed above, Engelbrecht disqualifies the purification of virus particles from cell cultures. There is of course no convincing reason for this disqualification, and it is therefore rightly ignored by practicing virologists. I will discuss this further below; here I would first like to give one example that should satisfy Engelbrecht’s demands. The studies in question concerned the hepatitis A virus, which had been detected in the stool samples of patients in the early 1970s [10]. Initially, it was not possible to grow this virus in cell cultures; however, it could be isolated from the stools of patients in considerable quantities.
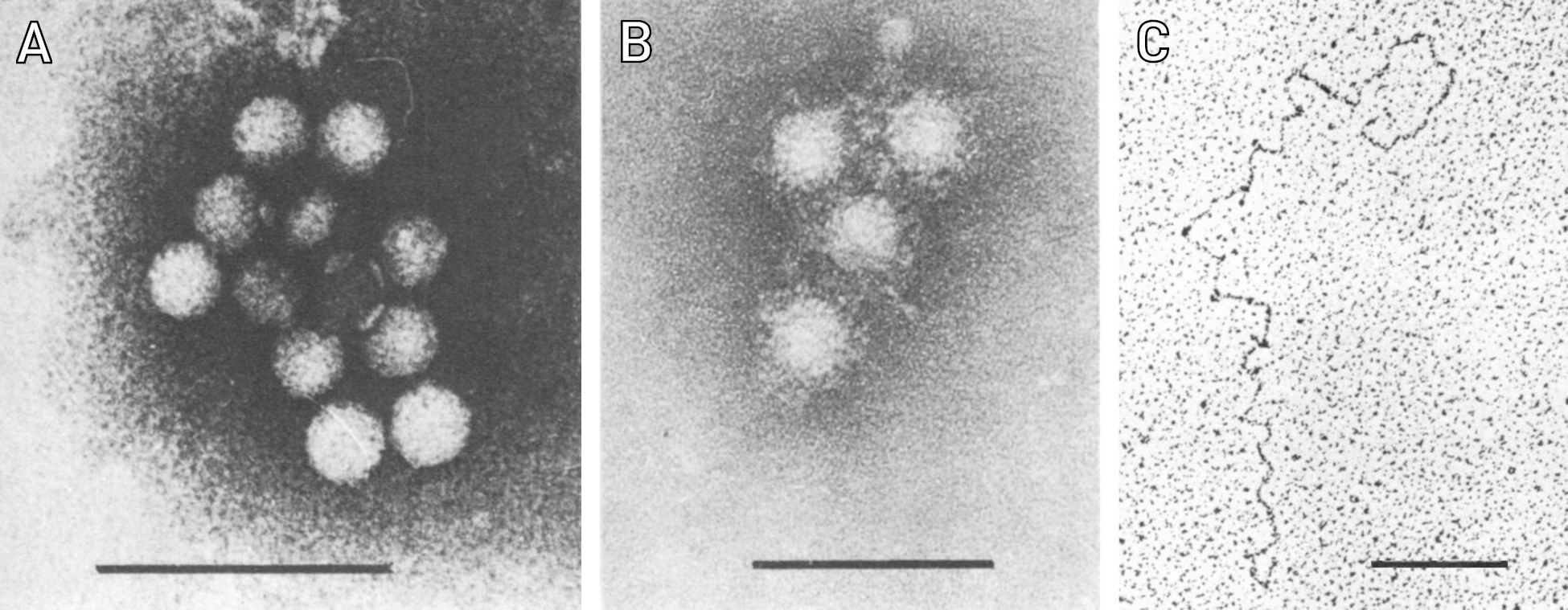
The virologists Siegl and Frösner carried out a series of detailed studies on such virus preparations. In their first work, they investigated the behavior of this virus by density gradient centrifugation [11]. This method was applied in two variations. One was used to determine the approximate size of the particles and the other to determine their density, i.e. their specific weight. Siegl and Frösner compared the hepatitis A virus directly with a known parvovirus and with the poliovirus. They found that the hepatitis A virus resembled the poliovirus and concluded that it must belong to the same virus family, namely the picornaviruses. This conclusion has held to this day. The authors also gave a detailed description of their purification protocol and showed electron micrographs of the purified particles (Figure 2). Electron microscopy was also used to determine the particle size more precisely than with centrifugation.
In their second study, the same authors examined the genomic nucleic acid contained in the virus particles [12]. They found that it consisted of single-stranded RNA. This finding fits the placement of the virus in the Picorna family. Finally, a third study involving two other authors investigated the composition of the protein molecules contained in the virus particles [13]. All three studies are very well and thoroughly documented and freely accessible.
These studies therefore meet all of Engelbrecht’s requirements: the particles were isolated from patient materials (stool samples), they were purified in a complex process and they were characterized using biophysical and biochemical methods as well as electron microscopy. It was also shown that the isolated viruses reacted with antibodies in the blood of hepatitis A, as would be expected from the causal pathogen.
The “refutation” of these studies by the virus skeptics will presumably simply consist of even more far-reaching demands—for example, that a battalion of volunteers should have been infected with the purified virus, regardless of the risk of severe disease; or that the viral RNA should also have been sequenced. As this sequence was missing, the skeptics were unfortunately forced to pronounce all other data presented invalid.
The genomic nucleotide sequence of the virus was determined somewhat later using a DNA copy of the genome generated in vitro and then propagated in bacteria [14]. And in 2014, a high-resolution structure of the entire virus particle was obtained using X-ray crystallography [15]—of course on samples that had been obtained in cell cultures. As already discussed at the beginning, every experimental study is limited by the technical possibilities of its period. The studies by Siegl and Frösner described here were as rigorous and thorough as was possible with the resources of the time. Nowadays, the sequence of the virus could even be determined directly from stool samples. Of course, such modern methods are once again anathema to virus skeptics.
5. On the “ban” on cell cultures by virus skeptics
The introduction of cell cultures for the isolation of viruses represented a major advance in virology. Their importance can be compared to that of solid culture media in bacteriology. Such culture media make it possible to separate mixed bacterial cultures and isolate the individual bacterial species they contain. In addition, the species can often be recognized or at least narrowed down based on the appearance of the bacterial colonies.
Viruses need host cells in order to grow. If the host cells are allowed to grow like a lawn on a smooth surface (covered by nutrient fluid), then individual virus particles can form visible plaques on such a lawn by gradually infecting other neighboring cells after initial multiplication in a single host cell. Such plaques are comparable to bacterial colonies on a solid culture medium. The progeny of a single virus particle can be isolated from a plaque; this can then be subcultured and propagated further. This process is not as simple and reliable as with bacterial colonies on solid culture media, but it is much simpler and more effective than would be possible in animal experiments.3
The infected cells in the plaques also often show morphological changes, so-called cytopathic effects. The appearance of such effects varies and may hint at the identity of the virus in question.
So if cell culture is such a useful method, why is it rejected by virus skeptics? As far as I can see, the following reasons are given:
- Cell cultures are “dirty” because the virus in them is not completely “pure”, i.e. free of other living matter.
- Cytopathic effects can be triggered not only by viruses, but also by other factors.
- One cannot be sure that a virus is the same after propagation in cell culture as it was before, or that it does not originate from the cultured cells themselves and not from the sample with which the culture was inoculated.
Of these three objections, the first two are nonsensical. Viruses can only multiply in living cells, so they can only ever be obtained from an “impure” environment. I assume readers need not be told whether cell cultures are less or more clean than, for example, sputum or stool samples. And that there are many ways to mishandle cell cultures will be clear to any practicing virologist, who will make sure to avoid them. I have never seen a laboratory working with cell cultures in which a non-inoculated culture was not carried along as a control in every experiment.
The third objection is not so easy to dismiss. There are indeed examples of cell cultures which had been assumed to be clean, but which themselves harbored viruses that then led to problems. One perhaps well-known example is the Marburg virus, which infected green monkeys that were used to obtain cell cultures. During this work, several laboratory workers became infected, some of whom died as a result. This incident occurred in 1967. Another example from the same period is polio vaccine batches that were contaminated with the potentially cancer-causing SV40 virus; this virus also originated from the cell cultures in question.
So this problem is real, but it is much more manageable nowadays. It is now easily possible to determine the complete genetic information present in an organism or cell line. In this way, one would also find any viral sequences present. In fact, a very large number of retroviruses and similar genetic elements have been found in the human genome [17]. In principle, such “resident” viruses could also produce virus particles in cell cultures, which would then contaminate other viruses cultivated in them. However, this could also be easily detected using modern methods. Moreover, this problem not only affects cells in culture, but also the cells of living humans and animals.4
It is also possible to experimentally establish that a virus cultivated in cell culture is identical to the virus in the original sample material. Specific antibodies as well as PCR and sequencing can be used for this purpose. In summary, it can be said that there are no substantial reasons for the “ban” imposed on cell cultures by virus skeptics. Practicing virologists will therefore continue to ignore this ban.
6. On the isolation and purification of SARS-CoV-2
Of all the virus skeptics’ theories, the most popular is probably that the SARS-CoV-2 virus has never been isolated. Of course, the virus skeptics base this claim entirely on their own self-made rules—namely that they will accept as evidence only those studies in which the virus was purified directly from patient materials—without the use of cell cultures—and then “further characterized and its genetic nature determined”, etc. As “proof” of their contention, they then present numerous exchanges of letters with virology laboratories and testing centers, in which the latter confirm that they did not follow the absurd rules imposed by the virus skeptics in anticipatory obedience.
To say it straight away—I am not aware of any study that meets all the demands of the virus skeptics. If a virus can be grown in cell culture, then it is clear that practicing virologists will not give up this option without need. But there are certainly studies in which the virus has been detected in patient material, then grown in cell culture and subsequently characterized in detail. One such study is that of Yao et al. [19]. Here, the virus was isolated from eleven individual patients using cell cultures, and each isolate was then sequenced. The other experiments reported in this paper are not relevant for the purpose of this article.
In the study by Liu et al. [20], the virus was isolated from a single patient. The sequence was only determined directly from the sample material in this case. The virus was grown in cell culture and its identity was verified by PCR. After further propagation in cell culture, it was inactivated by chemical treatment, purified by several centrifugation steps and finally examined by cryo-electron microscopy. The shape of the observed virus particles is typical for the coronavirus family (Figure 3).
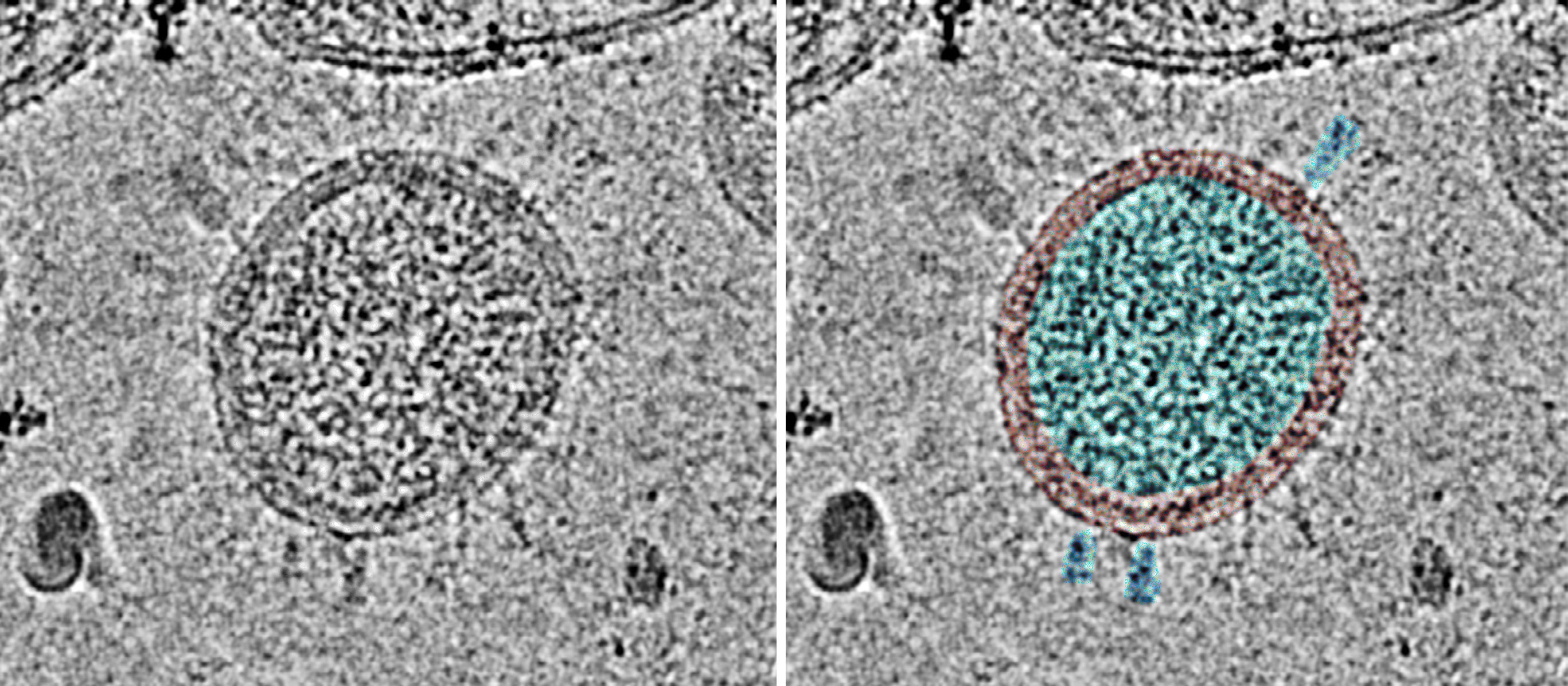
There are some further questions about SARS-CoV-2 which I will not go into here, in particular how reliable the determination of the sequence of the genome was. This topic is very technical and would go beyond the already wide scope of this article; and it is not germane to the central question of the existence of SARS-CoV-2. Nor do I intend to justify the mass misuse of PCR and other tests on clinically healthy people, or the grotesquely exaggerated panic that was fomented around this artificially induced “pandemic”. I merely claim that the existence of the SARS-CoV-2 virus has been sufficiently proven.
7. Conclusion
The idea that the existence of pathogenic viruses is “not proven” is nonsense. If you take it literally, then it is trivial, because in empirical science nothing can ever be proven once and for all. If you measure it by how well it agrees with the known observations, then it falls flat, as the few examples discussed here sufficiently show, which largely or at least partially conform to the rules stipulated by the virus skeptics.
But the fundamental issue is, of course, with those arbitrary restrictions that the virus skeptics want to impose on mainstream virologists. There are simply no rational arguments against the use of cell cultures for the detection of viruses, any more than there are against the use of solid culture media in bacteriology. The same applies to modern methods for the detection of viral nucleic acid sequences in cell cultures or in sample materials. Like all other methods, these can of course also be misused. Such misuse is objectionable, but a fundamental ban on the methods as such is unjustified.
The whole “proof procedure” devised by the virus skeptics has nothing to do with real science. Rather, it is reminiscent of the swimming test that used to be applied to those suspected of witchcraft. The limbs of the unfortunates were tied up and they were then lowered into the water; if they were still able to swim, their guilt was considered proven. If the virus skeptics have their way, then virologists should also submit to being tied up. If, contrary to expectations, they should still able to swim, this would certainly be ascribed to witchcraft.
I prefaced this article with a quote from Karl Popper. Another one fits here at the end: “The survival value of intelligence is that it allows us to extinct a bad idea, before the idea extincts us.” The “no virus” doctrine is nothing more than one of these bad ideas.
Notes
- I use the collective term “virus skeptics” here for all those who deny the existence of viruses or consider them “unproven”. (go back)
- Engelbrecht did not provide a citation, so I am unable to provide the English original of the quote. The text given here is back-translated from German. (go back)
- A study by Bohl et al. [16] illustrates the effort involved in isolating a single virus from two other initially present viruses when propagation is only possible in living animals. (go back)
- In this context, Engelbrecht claims that the treatment of cell cultures with antibiotics (to prevent bacterial contamination) could cause the cells to form “new gene sequences”, which “are not viral, mind you.” However, the study he cites as evidence [18] only speaks of the release of chromosomal and mitochondrial DNA; both are part of cellular DNA. There is no “formation of new gene sequences”. (go back)
References
The featured image was “borrowed” from this web page.
- (2024) Virusnachweis – wo bist Du?.
- (2024) Do viruses exist?.
- (1934) Die Logik der Forschung (Teuschner).
- (1982) `Über Tuberkulose.’ A tribute to Robert Koch’s discovery of the tubercle bacillus, 1882. Tubercle 63:3-17
- (1970) Recovery and characterization of a minute virus of canines. Infect. Immun. 1:503-8
- (1981) Isolation and characterization of feline rotavirus. J. Gen. Virol. 54:313-23
- (1986) Adsorption, purification, and growth characteristics of hepatitis A virus strain HAS-15 propagated in fetal rhesus monkey kidney cells. Journal of clinical microbiology 23:434-40
- (2024) “Gibt es überhaupt Viren?” Eine wissenschaftliche Analyse mit Sprengkraft.
- (1999) Zur Geschichte der frühen Virusforschung.
- (1973) Hepatitis A: detection by immune electron microscopy of a viruslike antigen associated with acute illness. Science 182:1026-8
- (1978) Characterization and classification of virus particles associated with hepatitis A. I. Size, density, and sedimentation. 377 26:40-7
- (1978) Characterization and classification of virus particles associated with hepatitis A. II. Type and configuration of nucleic acid. 377 26:48-53
- (1981) Characterization and classification of virus particles associated with hepatitis A. III. Structural proteins. J. Virol. 38:151-6
- (1985) Primary structure and gene organization of human hepatitis A virus. Proc. Natl. Acad. Sci. U. S. A. 82:2627-31
- (2014) Hepatitis A virus and the origins of picornaviruses. Nature 517:85-88
- (1982) Porcine pararotavirus: detection, differentiation from rotavirus, and pathogenesis in gnotobiotic pigs. 1861 15:312-9
- (2009) The impact of retrotransposons on human genome evolution. Nature reviews. Genetics 10:691-703
- (2017) Antibiotic-induced release of small extracellular vesicles (exosomes) with surface-associated DNA. Sci. Rep. 7:8202
- (2020) Patient-derived SARS-CoV-2 mutations impact viral replication dynamics and infectivity in vitro and with clinical implications in vivo. Cell Discov. 6:76
- (2020) The Architecture of Inactivated SARS-CoV-2 with Postfusion Spikes Revealed by Cryo-EM and Cryo-ET. Structure 28:1218-1224.e4